Engineers Make the Case for Waste to Energy
Engineers Make the Case for Waste to Energy
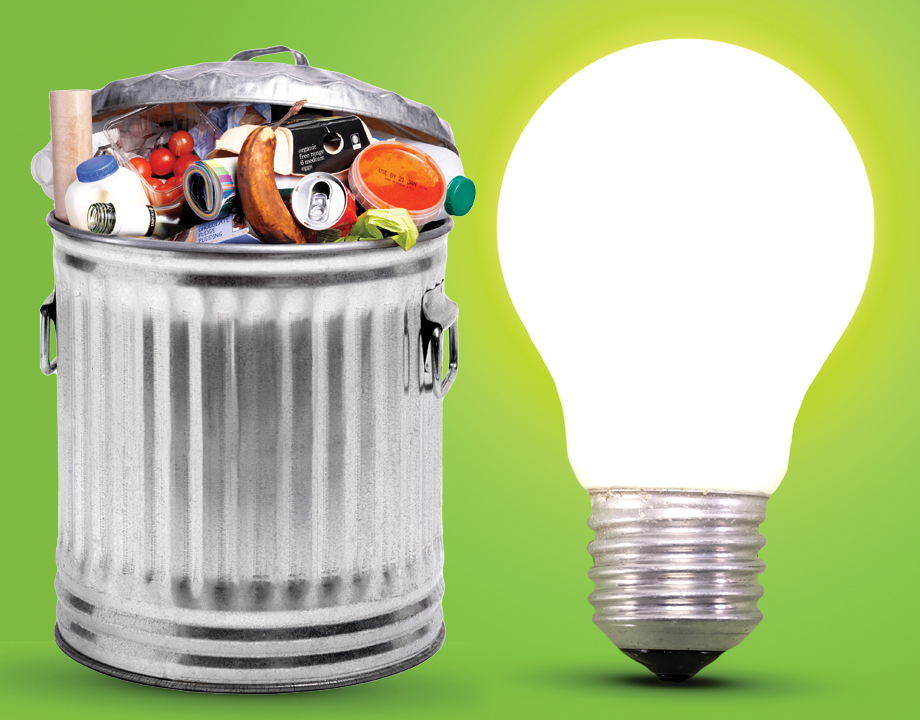
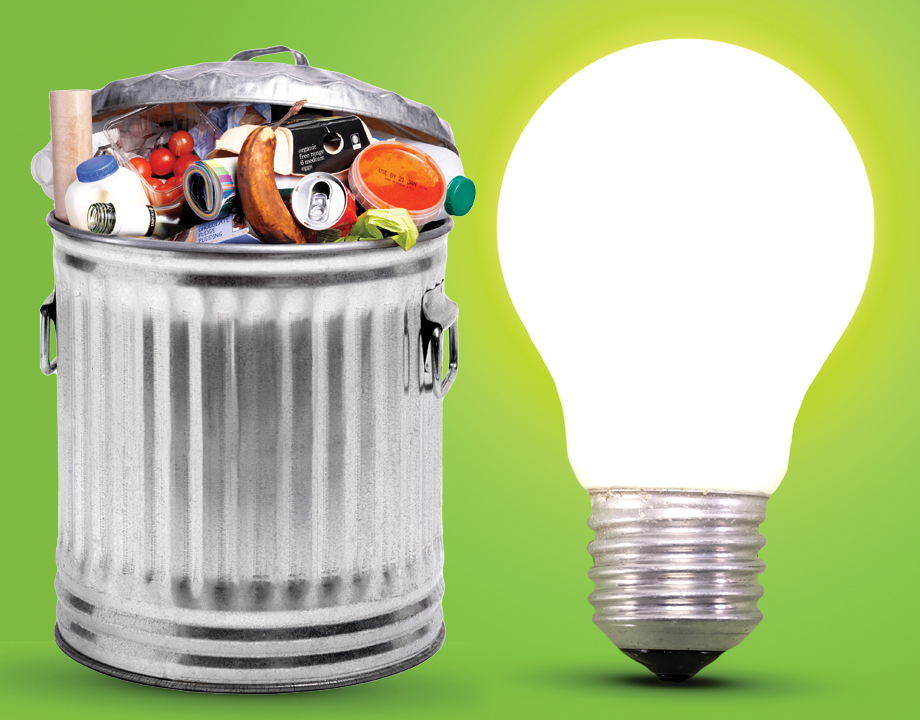
Americans produce more municipal solid waste than ever before. Instead of burying it, we should be turning it into low-carbon energy and recovering valuable materials.
Modern industry produces a vast amount of goods for consumption. While much of that is in the form of durable goods designed to last for years or decades, a great deal of what moves from the factory or port to the consumer has a relatively short life. Some, such as plastic packaging or food scraps, are thrown out very shortly after purchase.
The United States generated 292.4 million tons of municipal solid waste (MSW) in 2018 (the latest year with data available from the U.S. Environmental Protection Agency). That is around 5 pounds per person per day. According to the EPA, the largest categories of MSW by weight are paper and paperboard, food, yard trimmings, plastics, metals, wood, and textiles.
Much effort has been made to promote recycling of these materials, but to date only 69 million tons, less than one-quarter of MSW is recycled, mostly paper, metal, and glass. Another 43 million tons of material is composted. By far the most common waste management method is landfilling, with 146 million tons—more than half of all MSW.
Landfills have long been a problematic waste disposal solution with negative impact on groundwater and surrounding soil, but one emerging issue is their climate impact. MSW landfills are the third-largest source of methane emissions in the United States, accounting for approximately 15.1 percent of these emissions in 2019. The methane emissions from MSW landfills in 2019 were approximately equivalent to the annual CO2 emissions from the energy use of nearly 12.0 million homes. (Recent data and documentation suggest these landfill methane emissions are significantly underestimated.)
There is a better way of handling MSW that is not or cannot be recycled: thermal conversion, also called waste to energy. These facilities feed waste into a combustion chamber with air and incinerate it. The heat released from combustion produces steam for use in a district heat network, or to generate electricity, or do both in combined heat and power systems. In addition to enabling the heat content of the MSW to be recovered, combustion reduces its final volume by more than 90 percent, thus decreasing the need for landfills.
More on this Topic: Achieving Electricity Diversity Through Waste to Energy
Modern WTE facilities are nothing like the trash incinerators of old, which had limited pollution control and would release smoke across urban areas. In Western Europe, Japan, and elsewhere, waste to energy is the prominent management method, with some countries combusting up to 50 percent of their waste. In the U.S., however, only 12.8 percent of MSW is sent to WTE facilities. In fact, the U.S. is the only developed country where the number of WTE plants is declining. That seems to be due to a misunderstanding about the cost and environmental impact of these facilities.
We believe the advantages of WTE provide a robust case for expanding the role of WTE in the U.S.
According to the Energy Recovery Council, a trade group for the waste-to-energy industry, U.S. WTE plants process nearly 94,000 tons of MSW per day producing 2.5 GW of electricity. Combined heat and power plants generate an additional equivalent of 0.2 GW of thermal energy. The total amount of electricity from waste-to-energy plants is less than 1 percent of the total electricity consumption of the U.S. but, unlike wind or solar power, it is considered baseload power.
In terms of efficiency, a relatively new WTE facility in West Palm Beach, Fla., generates to the grid about 575 kWh of electric power per ton of MSW processed. That compares to coal (more than 1,900 kWh per ton) and other fuels. Similarly, the raw figures for carbon dioxide emissions are also poor; the EPA reports the burning of MSW for electricity produces 2,988 pounds of carbon dioxide per MWh, which would be around one-third more polluting than coal.
Those raw numbers obscure the full measure of WTE. For instance, the value reported by the EPA for MSW includes emissions for both the biogenic and fossil fractions of MSW. However, when considering CO2 emissions from MSW combustion, it is necessary to count only emissions from fossil fuel-based products such as plastics. The biogenic fraction of MSW is material, such as paper or food waste, generated from living organisms and is already in the planet’s carbon cycle. This biogenic fraction should not be included when determining the GHG outputs of combusting MSW for energy recovery.
Using the composition of MSW and the Energy Information Administration (EIA) methodology for allocating MSW to biogenic and non-biogenic energy, about 53 percent of the CO2 generated by MSW combustion facilities is from biogenic sources and 47 percent is derived from fossil sources. When factoring in only the fossil-derived waste, the CO2 emissions from WTE—1,016 pounds per MWh—is not only less than coal but also less that natural gas. Even this number may be an overestimate: One of us (Castaldi) recently published a manuscript reporting that the biogenic component of MSW is as high as 63 percent, which means the fossil emissions from WTE is lower than previous calculations. Further, by combusting the waste rather than landfilling and accounting for the reduced methane production from the waste, net GHG emissions are reduced even more.
Due to the high level of biogenic material in the waste stream, WTE is often considered to be a renewable energy source. For instance, the East Rockingham Waste to Energy Project near Perth, Australia, will handle 300,000 metric tons of waste per year and export 28.9 MW of electricity to the Western Australian grid when it becomes operational later this year. Due to the composition of the waste, more than 50 percent of the power is deemed to be renewable.
New technologies could reduce the net emissions even further. Advances have been made in the development of steel alloys that will enable WTE plants to operate at higher thermal efficiencies, which would mean more power produced for every pound of CO2 emissions. Also, Norway, Denmark, the Netherlands, and other countries have either begun trials of carbon capture systems on their WTE plants or have been making plans for adding such systems. Since WTE plants are smaller than coal-fired plants, the economics of adding carbon capture to them may have advantages. Such facilities could provide local sources of CO2 or be sited near saline aquifers that could offer the potential for sequestration.
Given the advantages of waste to energy, why does it have such a relatively low adoption rate in the United States? The decision to either combust or bury waste that cannot or is not recycled is influenced by constraints from local geological conditions, resource availability, legislative requirements, local social attitudes, and environmental impacts—but predominantly on economics. In general, in the U.S., treatment of MSW at WTE plants cost more than landfills, and revenues from disposal fees, sale of energy, and sales of recovered metals, rare earth minerals, and building materials have not been enough to make up for the extra cost.
When many of the existing WTE plants were built (particularly those built between 1980 and 2000) they relied upon and operated under the 1978 Public Utility Regulatory Policies Act (PURPA), which was implemented to promote energy conservation and the use of renewable energy, which at the time included WTE. Based on PURPA rates, those facilities were paid in the range of $0.08 to $0.12/kWh for the electrical power they produced. Those power purchase contacts have all expired, and WTE plants have had a difficult time competing in the energy markets.
Because of their long lead times needed to start up like fossil plants, WTE plants cannot participate in the lucrative peak power market, where gas turbines dominate. And with the low cost of electric generation from natural gas, wind, and solar power, the current electric wholesale prices being paid for electricity from WTE plants are reported to be as low as $0.02/kWh.
Each $0.01/kWh reduction in wholesale electricity price is the loss of approximately $5.50 per ton of MSW handled. One way to respond to lower revenue from energy sales is to increase tipping fees, which was already the largest source of revenue. The fees at most WTE plants range from $80 to $100 per ton, compared to around $55 per ton of MSW at landfills. (Landfill costs have significant regional variation; New York City pays about $120 per ton for waste disposal to distant landfills.) Also, while transportation costs (and transportation related emissions) are higher for most landfills compared to WTE plants, due to siting of landfills farther away from urban centers, those extra costs are not always reported in the final fee determination, which puts WTE at a further economic disadvantage.
Editor's Choice: Turning E-Waste into an Opportunity
It has therefore been difficult to raise tipping fees high enough to make up for the loss in energy sales. By contrast, many of European countries and countries like Australia have imposed high landfill fees (as high as $100 per ton) to discourage landfilling, which improves the competitiveness of waste to energy.
Due to the inability for WTE plant operators to successfully replace that lost revenue, WTE plants in the U.S. have been closing. According to the Energy Recovery Council, there were 97 WTE facilities in the U.S. in 2001, and by 2018 only 75 remained.
The decline in waste-to-energy facilities in the U.S. is not inevitable and is not seen elsewhere in the world. In contrast with the situation in the U.S., there are 410 WTE facilities in Europe with several more under construction or being planned. From 2011 to 2015, the WTE capacity of China more than doubled to 339 plants. Countries such as Ethiopia have recently installed a WTE facility to sustainably manage their waste.
The key to restoring and expanding WTE in the U.S. is viewing it as a solution with many interrelated advantages such as metals and minerals recovery. While it isn’t the cheapest option for waste disposal or energy production, it offers other unique benefits, simultaneously.
For instance, WTE plants are an integral part of emergency management. In the aftermath of large-scale weather disasters such as Superstorm Sandy and Hurricane Irma, vast amounts of household and other debris had to be disposed in a short amount of time. In the Northeast and in Florida, WTE plants provided an environmentally safe disposal of mold contaminated material due to the high temperatures achieved during processing, which is not present in landfills.
Those high temperatures also allow WTE plants to provide for destruction of organics, bacteria, and viruses. Diverting them from landfills and destroying them in WTE plants produces a health and environmental benefit that is hard to account for in most economic analyses.
As for energy production, WTE plants are baseload power plants rather than intermittent sources such as wind and solar. They also have annual capacity factors of 85 percent or greater. This is in sharp contrast with other renewable power sources with capacity factors in the 15-percent to 45-percent range. What’s more, WTE plants are usually sited in or close to the urban centers that produce MSW. That’s an advantage over power plants and wind turbines that are often hundreds of miles away and enable them to provide good voltage stabilization and a reliable and dependable source of energy.
Also, due to their always-on capability, power from WTE facilities could be used in periods of electricity surplus, such as very windy nights or very sunny weekend afternoons, to provide renewable power for energy storage systems or hydrogen generation plants.
More by This Author: Catalyzing a Solution for Plastic Waste
Keeping existing WTE plants running helps improve quality of life in cities and suburbs. Landfills close to cities are filling up and shutting down. When WTE facilities close as well, waste must be hauled to more distant landfills, increasing transportation costs, emissions, and road congestion and potentially exposing systems to cascading disruptions that create waste backups at key hubs or cities.
As technology improves, the next generation of waste-to-energy plants will be more efficient and recover more energy and materials.
A 2019 report from the DOE’s Office of Energy Efficiency and Renewable Energy, Waste-to-Energy from Municipal Solid Wastes, identified some opportunities to improve the economics of WTE facilities. These include implementation of more advanced feedstock characterization and pretreatments to selectively remove problematic constituents and provide a more homogenous fuel for the incinerators. It also identified use of new, less expensive materials (including ceramics) that could abate corrosion and fouling and development of new catalysts that could reduce nitrogen oxides at lower temperatures as ways to diminish incinerator costs. New ash recycling technologies in development would increase metal and rare-earth mineral recovery, which would create an enhanced revenue stream.
By providing the dual benefit of delivering a low carbon source of power generation and providing an environmentally sustainable management of municipal waste, WTE facilities provide a valuable solution to two vitally important parts of the infrastructure. Given that the amount of waste and the demand for energy will only increase in the coming decades, the United States should be following the lead of other countries and expanding its use of this versatile tool.
Marco J. Castaldi is director of both the Earth System Science and Environmental Engineering Program and the Earth Engineering Center at the City College of New York, as well as a professor of chemical engineering there. Jeff LeBlanc, P.E., is a product design engineer at Advance Products and Systems in Lafayette, La. Anthony Licata is an ASME Fellow and partner at Licata Energy & Environmental Consultants, Inc. in Yonkers, N.Y.
The authors are all members of ASME’s Material & Energy Recovery Division, and LeBlanc is the chairman.
To learn more:
• “A review of LCA assumptions impacting whether landfilling or incineration results in less greenhouse gas emissions,” by M. Anshassi, H. Sackles, and T.G. Townsend Resources, Conservation and Recycling, Volume 174, Nov. 2021, 105810.
• “Waste-to-Energy Benefits,” ASME Material & Energy Recovery Division white paper, July 2021.
• “Waste-To-Energy From Municipal Solid Wastes,” EERE report, August 2019.
• “Perspectives on Energy Recovery from U.S. Plastic Waste.” D. Peck, Power Magazine, 2 Aug., 2021.
The United States generated 292.4 million tons of municipal solid waste (MSW) in 2018 (the latest year with data available from the U.S. Environmental Protection Agency). That is around 5 pounds per person per day. According to the EPA, the largest categories of MSW by weight are paper and paperboard, food, yard trimmings, plastics, metals, wood, and textiles.
Much effort has been made to promote recycling of these materials, but to date only 69 million tons, less than one-quarter of MSW is recycled, mostly paper, metal, and glass. Another 43 million tons of material is composted. By far the most common waste management method is landfilling, with 146 million tons—more than half of all MSW.
Landfills have long been a problematic waste disposal solution with negative impact on groundwater and surrounding soil, but one emerging issue is their climate impact. MSW landfills are the third-largest source of methane emissions in the United States, accounting for approximately 15.1 percent of these emissions in 2019. The methane emissions from MSW landfills in 2019 were approximately equivalent to the annual CO2 emissions from the energy use of nearly 12.0 million homes. (Recent data and documentation suggest these landfill methane emissions are significantly underestimated.)
There is a better way of handling MSW that is not or cannot be recycled: thermal conversion, also called waste to energy. These facilities feed waste into a combustion chamber with air and incinerate it. The heat released from combustion produces steam for use in a district heat network, or to generate electricity, or do both in combined heat and power systems. In addition to enabling the heat content of the MSW to be recovered, combustion reduces its final volume by more than 90 percent, thus decreasing the need for landfills.
More on this Topic: Achieving Electricity Diversity Through Waste to Energy
Modern WTE facilities are nothing like the trash incinerators of old, which had limited pollution control and would release smoke across urban areas. In Western Europe, Japan, and elsewhere, waste to energy is the prominent management method, with some countries combusting up to 50 percent of their waste. In the U.S., however, only 12.8 percent of MSW is sent to WTE facilities. In fact, the U.S. is the only developed country where the number of WTE plants is declining. That seems to be due to a misunderstanding about the cost and environmental impact of these facilities.
We believe the advantages of WTE provide a robust case for expanding the role of WTE in the U.S.
Emissions in Perspective
According to the Energy Recovery Council, a trade group for the waste-to-energy industry, U.S. WTE plants process nearly 94,000 tons of MSW per day producing 2.5 GW of electricity. Combined heat and power plants generate an additional equivalent of 0.2 GW of thermal energy. The total amount of electricity from waste-to-energy plants is less than 1 percent of the total electricity consumption of the U.S. but, unlike wind or solar power, it is considered baseload power.
In terms of efficiency, a relatively new WTE facility in West Palm Beach, Fla., generates to the grid about 575 kWh of electric power per ton of MSW processed. That compares to coal (more than 1,900 kWh per ton) and other fuels. Similarly, the raw figures for carbon dioxide emissions are also poor; the EPA reports the burning of MSW for electricity produces 2,988 pounds of carbon dioxide per MWh, which would be around one-third more polluting than coal.
Those raw numbers obscure the full measure of WTE. For instance, the value reported by the EPA for MSW includes emissions for both the biogenic and fossil fractions of MSW. However, when considering CO2 emissions from MSW combustion, it is necessary to count only emissions from fossil fuel-based products such as plastics. The biogenic fraction of MSW is material, such as paper or food waste, generated from living organisms and is already in the planet’s carbon cycle. This biogenic fraction should not be included when determining the GHG outputs of combusting MSW for energy recovery.
Using the composition of MSW and the Energy Information Administration (EIA) methodology for allocating MSW to biogenic and non-biogenic energy, about 53 percent of the CO2 generated by MSW combustion facilities is from biogenic sources and 47 percent is derived from fossil sources. When factoring in only the fossil-derived waste, the CO2 emissions from WTE—1,016 pounds per MWh—is not only less than coal but also less that natural gas. Even this number may be an overestimate: One of us (Castaldi) recently published a manuscript reporting that the biogenic component of MSW is as high as 63 percent, which means the fossil emissions from WTE is lower than previous calculations. Further, by combusting the waste rather than landfilling and accounting for the reduced methane production from the waste, net GHG emissions are reduced even more.
Due to the high level of biogenic material in the waste stream, WTE is often considered to be a renewable energy source. For instance, the East Rockingham Waste to Energy Project near Perth, Australia, will handle 300,000 metric tons of waste per year and export 28.9 MW of electricity to the Western Australian grid when it becomes operational later this year. Due to the composition of the waste, more than 50 percent of the power is deemed to be renewable.
New technologies could reduce the net emissions even further. Advances have been made in the development of steel alloys that will enable WTE plants to operate at higher thermal efficiencies, which would mean more power produced for every pound of CO2 emissions. Also, Norway, Denmark, the Netherlands, and other countries have either begun trials of carbon capture systems on their WTE plants or have been making plans for adding such systems. Since WTE plants are smaller than coal-fired plants, the economics of adding carbon capture to them may have advantages. Such facilities could provide local sources of CO2 or be sited near saline aquifers that could offer the potential for sequestration.
Cost Crunch
Given the advantages of waste to energy, why does it have such a relatively low adoption rate in the United States? The decision to either combust or bury waste that cannot or is not recycled is influenced by constraints from local geological conditions, resource availability, legislative requirements, local social attitudes, and environmental impacts—but predominantly on economics. In general, in the U.S., treatment of MSW at WTE plants cost more than landfills, and revenues from disposal fees, sale of energy, and sales of recovered metals, rare earth minerals, and building materials have not been enough to make up for the extra cost.
When many of the existing WTE plants were built (particularly those built between 1980 and 2000) they relied upon and operated under the 1978 Public Utility Regulatory Policies Act (PURPA), which was implemented to promote energy conservation and the use of renewable energy, which at the time included WTE. Based on PURPA rates, those facilities were paid in the range of $0.08 to $0.12/kWh for the electrical power they produced. Those power purchase contacts have all expired, and WTE plants have had a difficult time competing in the energy markets.
Because of their long lead times needed to start up like fossil plants, WTE plants cannot participate in the lucrative peak power market, where gas turbines dominate. And with the low cost of electric generation from natural gas, wind, and solar power, the current electric wholesale prices being paid for electricity from WTE plants are reported to be as low as $0.02/kWh.
Each $0.01/kWh reduction in wholesale electricity price is the loss of approximately $5.50 per ton of MSW handled. One way to respond to lower revenue from energy sales is to increase tipping fees, which was already the largest source of revenue. The fees at most WTE plants range from $80 to $100 per ton, compared to around $55 per ton of MSW at landfills. (Landfill costs have significant regional variation; New York City pays about $120 per ton for waste disposal to distant landfills.) Also, while transportation costs (and transportation related emissions) are higher for most landfills compared to WTE plants, due to siting of landfills farther away from urban centers, those extra costs are not always reported in the final fee determination, which puts WTE at a further economic disadvantage.
Editor's Choice: Turning E-Waste into an Opportunity
It has therefore been difficult to raise tipping fees high enough to make up for the loss in energy sales. By contrast, many of European countries and countries like Australia have imposed high landfill fees (as high as $100 per ton) to discourage landfilling, which improves the competitiveness of waste to energy.
Due to the inability for WTE plant operators to successfully replace that lost revenue, WTE plants in the U.S. have been closing. According to the Energy Recovery Council, there were 97 WTE facilities in the U.S. in 2001, and by 2018 only 75 remained.
Versatile Solution
The decline in waste-to-energy facilities in the U.S. is not inevitable and is not seen elsewhere in the world. In contrast with the situation in the U.S., there are 410 WTE facilities in Europe with several more under construction or being planned. From 2011 to 2015, the WTE capacity of China more than doubled to 339 plants. Countries such as Ethiopia have recently installed a WTE facility to sustainably manage their waste.
The key to restoring and expanding WTE in the U.S. is viewing it as a solution with many interrelated advantages such as metals and minerals recovery. While it isn’t the cheapest option for waste disposal or energy production, it offers other unique benefits, simultaneously.
For instance, WTE plants are an integral part of emergency management. In the aftermath of large-scale weather disasters such as Superstorm Sandy and Hurricane Irma, vast amounts of household and other debris had to be disposed in a short amount of time. In the Northeast and in Florida, WTE plants provided an environmentally safe disposal of mold contaminated material due to the high temperatures achieved during processing, which is not present in landfills.
Those high temperatures also allow WTE plants to provide for destruction of organics, bacteria, and viruses. Diverting them from landfills and destroying them in WTE plants produces a health and environmental benefit that is hard to account for in most economic analyses.
As for energy production, WTE plants are baseload power plants rather than intermittent sources such as wind and solar. They also have annual capacity factors of 85 percent or greater. This is in sharp contrast with other renewable power sources with capacity factors in the 15-percent to 45-percent range. What’s more, WTE plants are usually sited in or close to the urban centers that produce MSW. That’s an advantage over power plants and wind turbines that are often hundreds of miles away and enable them to provide good voltage stabilization and a reliable and dependable source of energy.
Also, due to their always-on capability, power from WTE facilities could be used in periods of electricity surplus, such as very windy nights or very sunny weekend afternoons, to provide renewable power for energy storage systems or hydrogen generation plants.
More by This Author: Catalyzing a Solution for Plastic Waste
Keeping existing WTE plants running helps improve quality of life in cities and suburbs. Landfills close to cities are filling up and shutting down. When WTE facilities close as well, waste must be hauled to more distant landfills, increasing transportation costs, emissions, and road congestion and potentially exposing systems to cascading disruptions that create waste backups at key hubs or cities.
As technology improves, the next generation of waste-to-energy plants will be more efficient and recover more energy and materials.
A 2019 report from the DOE’s Office of Energy Efficiency and Renewable Energy, Waste-to-Energy from Municipal Solid Wastes, identified some opportunities to improve the economics of WTE facilities. These include implementation of more advanced feedstock characterization and pretreatments to selectively remove problematic constituents and provide a more homogenous fuel for the incinerators. It also identified use of new, less expensive materials (including ceramics) that could abate corrosion and fouling and development of new catalysts that could reduce nitrogen oxides at lower temperatures as ways to diminish incinerator costs. New ash recycling technologies in development would increase metal and rare-earth mineral recovery, which would create an enhanced revenue stream.
By providing the dual benefit of delivering a low carbon source of power generation and providing an environmentally sustainable management of municipal waste, WTE facilities provide a valuable solution to two vitally important parts of the infrastructure. Given that the amount of waste and the demand for energy will only increase in the coming decades, the United States should be following the lead of other countries and expanding its use of this versatile tool.
Marco J. Castaldi is director of both the Earth System Science and Environmental Engineering Program and the Earth Engineering Center at the City College of New York, as well as a professor of chemical engineering there. Jeff LeBlanc, P.E., is a product design engineer at Advance Products and Systems in Lafayette, La. Anthony Licata is an ASME Fellow and partner at Licata Energy & Environmental Consultants, Inc. in Yonkers, N.Y.
The authors are all members of ASME’s Material & Energy Recovery Division, and LeBlanc is the chairman.
To learn more:
• “A review of LCA assumptions impacting whether landfilling or incineration results in less greenhouse gas emissions,” by M. Anshassi, H. Sackles, and T.G. Townsend Resources, Conservation and Recycling, Volume 174, Nov. 2021, 105810.
• “Waste-to-Energy Benefits,” ASME Material & Energy Recovery Division white paper, July 2021.
• “Waste-To-Energy From Municipal Solid Wastes,” EERE report, August 2019.
• “Perspectives on Energy Recovery from U.S. Plastic Waste.” D. Peck, Power Magazine, 2 Aug., 2021.